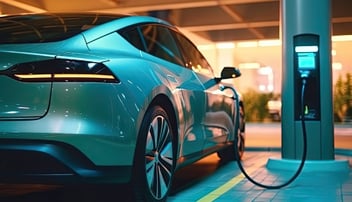
Most people are familiar with the concept of melting point — the temperature at which a solid changes into a liquid state. But not all materials have a defined melting point. An amorphous polymer has a glass transition temperature, which is not a..
Most people are familiar with the concept of melting point — the temperature at which a solid changes into a liquid state. But not all materials have a defined melting point. An amorphous polymer has a glass transition temperature, which is not a single temperature at all but a range of temperatures across which the properties of the polymer change dramatically.
Ever wondered why house painters talk so much about application temperatures? It’s not just because they like to work on so-called Goldilocks days, when it’s not too hot and not too cold. It’s because the paint they’re using — most likely a latex-based formula — performs best when it’s applied between 10° to 20°C (50° and 70°F). If they paint outside of this range of temperatures, the paint may not cure properly, which means it won’t adhere completely to the surface and could even crack or peel.
This property of a latex paint arises because latex binders are classified as amorphous polymers, and all amorphous polymers have a fundamental feature known as the glass transition temperature, or Tg. The glass transition temperature affects how a finished polymer will behave, so it’s extremely important.
In this article, we’re going to explore the ins and outs of Tg: what it is, how it’s measured, and how it’s used to modify the properties of a finished product. Let’s begin by discussing amorphous polymers and how they differ from their crystalline counterparts.
Amorphous vs. Crystalline Polymers
There are many kinds of polymers, including plastics, rubber, elastomers and latexes, but they all have one thing in common — they contain long-chain molecules organized into complex arrangements. In some polymers, classified as crystalline, the long chains of molecules arrange themselves in an orderly fashion, often with one molecular chain locked against another. In other polymers, classified as amorphous, the long chains of molecules are randomly packed together and show no discernible order.
When crystalline polymers are heated, they reach a certain temperature at which the orderly arrangement of their long-chain structure transitions to a random and disorganized arrangement. The polymer literally transitions from a solid to a liquid. This temperature is known as the melting point, or Tm. The melting point of polypropylene, for example, is 175°C (347°F). For high-density polyethylene, it’s 130°C (266°F).
When amorphous polymers are heated, they don’t melt suddenly. Instead, they reach a range of temperatures over which the material becomes less glassy and more rubber-like or vice versa. As a result, amorphous polymers don’t have a melting point — they have a glass transition temperature, or Tg. The glass transition temperature of a specific polymer may be listed as a single temperature, but this number is an averaged value calculated from a range of temperatures. We’ll explore this more in the next section.
It should also be noted that some polymers can have a portion of their chains that are amorphous and a portion that are crystalline. These polymers would have both a melting point and a glass transition temperature.
Measuring Tg
Chemists use several methods to measure the glass transition temperature of amorphous polymers, but the most common is differential scanning calorimetry, or DSC. A calorimeter is a piece of equipment that measures quantities of absorbed or emitted heat in a sample placed in the device. In DSC, the polymer sample is placed in the device and cooled from room temperature to a certain temperature, let’s say -100°C as an example. Next, it is heated from -100°C to 50°C, or a similar temperature, at a steady rate.
A computer attached to the system measures the thermal response of the polymer, producing a plot of heat flow versus temperature, similar to the one shown below. The glass transition appears as a “kink” in the plot. The glass transition temperature is calculated using a half-height technique, which means identifying the midpoint of the “kink” or the middle of the sloped region.
To understand why a heated amorphous polymer behaves in this fashion, it’s helpful to consider what’s happening at the molecular level. Remember, the long chains of amorphous polymers are randomly packed together, but in the glassy state (that is, below Tg), the bonds in the chains have little segmental motion, which makes the material harder and more brittle. At Tg, as the thermal energy increases, the bonds gain more segmental motion, and the chains begin sliding past one another at a noticeable rate. As this happens, the polymer becomes less glassy and more flexible.
Now let’s turn our attention to the role Tg plays when developing latex binders for specific applications.
The Importance of Tg
The transition from the glassy state to the rubbery state is accompanied by dramatic changes in the physical properties of amorphous polymers, so by choosing a polymer based on its Tg, it’s possible to select how you want a polymer to behave for certain end-use applications. For example, a polymer with a low Tg tends to feel soft and pliable or even sticky (tacky) at room temperature. A polymer with a Tg close to room temperature tends to feel tough and leathery. And a polymer with a high Tg tends to be hard and brittle at room temperature. The table below shows the glass transition temperatures for several important homopolymers — polymers made of a single species of monomer.
Monomer | Polymer Tg (°C) | Rating |
Butadiene | -85 | Soft |
2-Ethylhexyl Acrylate | -60 | Soft |
n-Butyl Acrylate | -54 | Soft |
Ethyl Acrylate | -23 | Soft |
Methyl Acrylate | +8 | Middle |
Room Temperature: 20-25°C | ||
Vinyl Acetate | +28 | Middle |
Vinyl Chloride | +75 | Hard |
Acrylonitrile | +97 | Hard |
Styrene | +100 | Hard |
Methyl Methacrylate | +105 | Hard |
For copolymers — that is, polymers made from two species of monomers — Tg is slightly more complicated. That’s because Tg can be manipulated by varying the polymer composition. For example, in a styrene-butadiene latex emulsion, the styrene has a Tg of 100°C while that of butadiene is -85°C. Changing the ratio of styrene to butadiene will change the Tg.
If we consider the styrene-butadiene latex binders developed by Mallard Creek Polymers, the impact of chemical composition on Tg becomes evident. For example, a carboxylated styrene-butadiene emulsion with a Tg of -56°C might be very soft at room temperature and ideally suited for pressure sensitive adhesives. Another general-purpose styrene-butadiene polymer might have a higher Tg of -15°C, giving the product a low stiffness and helping it to form a soft but tough film.
Tg and Minimum Film Formation Temperature
When it comes to paints, coatings and adhesives, applications that rely on a stable latex film to form, chemists also discuss something known as the minimum film formation temperature (MFFT). MFFT refers to the lowest temperature at which a latex will uniformly coalesce when laid on a substrate as a thin film. MFFT is typically close to the glass transition temperature of a polymer, though chemists are always striving to strike the right balance between proper film formation and hardness. Many coating-grade polymers are specially designed to have a lower MFFT compared to Tg in order to impart better film formation while maintaining its final level of strength.
All of which brings us back to the house painters we discussed at the beginning of the article. They may not know the terms glass transition temperature or minimum film formation temperature, but they rely on the principles every day. At Mallard Creek Polymers, we also rely on Tg to understand how a latex will perform at the range of temperatures the product will encounter. Our specialists can work with your team to understand your application needs and design a formula that provides superior performance in any environment. Contact us today to start a conversation.
Be sure to check out the other articles in our Latex Binders 101 series:
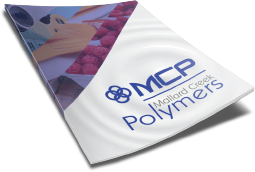