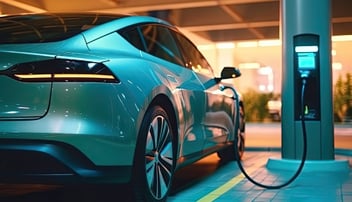
Polymers are unlike other types of materials because of their high molecular weight. Molecular weight is the value used to express the size of a molecule. Water, for example, has a molecular weight of 18 atomic mass units. Polymers are much larger,..
Polymers are unlike other types of materials because of their high molecular weight. Molecular weight is the value used to express the size of a molecule. Water, for example, has a molecular weight of 18 atomic mass units. Polymers are much larger, with molecular weights ranging from tens of thousands up to several million atomic mass units. The sheer size of polymers has a great impact on their unique properties.
One important implication of polymer size is how atoms behave in different phase states. Smaller-molecule compounds have three states of matter — solids, liquids and gases. The particles of a solid are packed closely together and have very little motion. The particles of a liquid, by comparison, are more loosely packed and slide more easily past one another. And the particles of a gas are very loosely packed and move about with great energy.
Large molecules, like polymers, behave differently because there are so many more atoms to interact with each other. For example, polymers aren’t thought of simply as solids — they are classified further into two types: amorphous and crystalline. What’s more, some polymeric solids can be both amorphous and crystalline at the same time. This surprising idea is particularly important to the study of polymers. In this article, we’ll explain the difference between amorphous and crystalline polymers and provide some common examples of each.
Polymer Morphology
In polymer chemistry, morphology is a key factor in describing the distinction between amorphous and crystalline solids. Polymers with an amorphous morphology have their atoms held together in a loose structure, but this structure is never orderly or predictable, which is why chemists will say that amorphous solids have no long-range order.
To understand this better, think of a polymer chain as a piece of spaghetti. A piece of uncooked spaghetti forms a nice straight chain, but if you cook it in boiling water, the chain softens and becomes capable of complex folding. Cook an entire box of spaghetti and place the noodles in a strainer, and you end up with a wildly random pile of intertwined “polymers.”
Chemists call this chain entanglement, and in this configuration, there is no long-range order — the chains are arrayed randomly throughout the material, making atomic positions quasi-random. This describes how amorphous polymers behave, and they would demonstrate the properties of any other amorphous, or glass-like, solid.
In crystalline polymers, the chains behave differently. They still form folds, but instead of becoming hopelessly tangled, they form orderly stacks of folded chains, known as lamellae. Lamellae bring long-range order to polymers, which is more like the orderly arrangement of atoms in typical crystals. Interestingly, some lamellae in certain polymers have small numbers of chains that loop out from the orderly stacks, much like a few wild hairs sticking up from a nicely groomed hairdo. This creates amorphous regions in an otherwise crystalline polymer. We’ll look at the implications of this in the next section.
Degree of Crystallinity
Most crystalline polymers have amorphous regions, which means crystalline polymers are never completely crystalline. Scientists often refer to a polymer’s degree of crystallinity to describe where it sits along this spectrum. Crystallinity can range from 0 percent (entirely amorphous) to 100 percent (entirely crystalline), but most polymers fall somewhere between those extremes. Chain flexibility — both flexing along the entire chain and flexing in bonds between atoms — plays a big role in polymer crystal formation. As chains flex and bend against each other, various attractive and repulsive forces affect how polymer chains arrange themselves, either more orderly or less orderly.
Degree of crystallinity is directly related to whether a polymer melts like a typical solid or whether it transitions between glassy and rubbery states. Highly crystalline polymers have a more traditional melting point, so when they are heated, they reach a certain temperature at which the orderly arrangement of their long-chain structure transitions to a random and disorganized arrangement. This value is usually a specific number, designated as the melting point, or Tm.
Amorphous solids don’t melt suddenly when they’re heated. Instead, they reach a range of temperatures over which the material becomes less glassy and more rubber-like or vice versa. As a result, amorphous polymers don’t have a melting point — they have a glass transition temperature, or Tg. The glass transition temperature of a specific polymer may be listed as a single temperature, but this number is a representative value representing a range of temperatures.
To explain glass transition temperature in terms of molecular motion, we would say that, at temperatures below Tg, the amorphous polymer chains cannot rotate or move in space (the cooked spaghetti is frozen and cannot move). This produces the glassy state, which is hard, rigid, and brittle. When the temperature rises above Tg, the entangled chains can move (small portions of the spaghetti noodles can move around). This produces a rubbery state, when an amorphous polymer is soft and flexible.
Now let’s look at some examples of crystalline and amorphous polymers to examine properties of real-world materials.
CAN'T FIND WHAT YOU'RE LOOKING FOR?
Examples of Amorphous and Crystalline Polymers
Although 100 percent and 0 percent crystallinity are rare, some polymers fall close to either extreme. Those that tend toward high crystallinity are rigid, have high melting points, and are less affected by solvent penetration. Those that tend toward high amorphousness are softer, have glass transition temperatures, and are penetrated more by solvents than are their crystalline counterparts. Here are some examples, along with their key properties:
Crystalline Polymers |
||||
Nylon![]() |
Polypropylene |
Polyethylene |
Polystyrene |
|
Monomer(s): | adipic acid & hexamethylene diamine | propylene | ethylene | styrene |
Tm: | 260°C (500°F) | 174°C (345°F) | 137°C (279°F) | 270°C (518°F) |
Uses: | thermoplastics, fibers | thermoplastics, fibers | thermoplastics, fibers | thermoplastics |
Polymerization: | acid catalyzed condensation polymerization | Zieglar-Natta polymerization | free radical chain polymerization | metallocene catalysis polymerization |
Amorphous Polymers |
||||
Polypropylene (Atactic*) ![]() |
Poly(methyl methacrylate)![]() |
Polybutadiene![]() |
Polystyrene (Atactic*) ![]() |
|
Monomer(s): | propylene | methyl methacrylate | butadiene | styrene |
Tg: | -17°C (1.4°F) | 120°C (248°F) | -106°C (-159°F) | 100°C (212°F) |
Uses: | elastomers | thermoplastics | tires, belts, hoses, gaskets, and seals | thermoplastics |
Polymerization: | Metallocene catalysis polymerization | free radical vinyl polymerization | Ziegler-Natta polymerization, free radical polymerization | free radical vinyl polymerization |
*Notice that certain polymers appear in both the crystalline and amorphous categories. To understand why, it helps to realize that polymers can have multiple configurations. A polymer has a main backbone with small clusters of atoms, called pendant groups, coming off of the chain. If all of the pendant groups are on the same side of the chain, the polymer is isotactic. If the pendant groups come on alternating sides of the chain, the polymer is said to be syndiotactic. If the pendant groups are on both sides, but in no particular order, the polymer is atactic. These structural differences can have a significant impact on the properties of a polymer.
Amorphous Polymer Specialists
Many applications of polymers and polymer coatings need flexibility at low to ambient temperatures. That’s where amorphous polymers are the right choice. Conversely, when hardness and rigidity are required, a polymer with greater crystallinity may be preferred. Mallard Creek Polymers specializes in the design and manufacturing of amorphous polymers. We work with customers to understand their needs and to develop emulsion polymers that address those needs. Call us for more information.
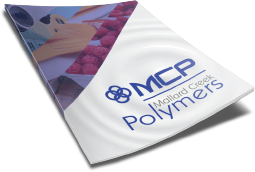